A single spin is the smallest possible magnetic field sensor, capable of mapping magnetisation with quantum-limited sensitivity and nanoscale spatial resolution. Electronic spins associated to the nitrogen-vacancy (NV) colour centre in diamond are widely used as nanoscale quantum sensors in a variety of geometries (scanning probe microscopes, widefield sensing, etc) over a wide temperature range. Our work in this area is related to the optimisation of the sensor capabilities, in particular with nanoscale magnetic resonance in mind, and to its application to study many-body physics and magnetic ordering in 2D materials.
Real-time adaptive optimisation and machine learning. One of the main bottlenecks for NV magnetometry is the data acquisition time: especially for long dynamical decoupling sequences with high spectral selectivity, required in nanoscale magnetic resonance, the signal acquisition timescales become prohibitively long. Our solution is to add self-optimisation capabilities to our sensor, exploiting Bayesian algorithms that enable optimising measurement settings in real-time based on previous outcomes. We implement these type of protocols using real-time microcontrollers, FPGAs and programmable arbitrary waveform generators.
In addition, we are developing automated learning algorithms to extract information from the environment. This is particularly important in nanoscale magnetic resonance, where the single-electron sensor detects simultaneously signals from multiple individual nuclear spins that need to be isolated and identified. For this purpose, we use both deep learning and Markov-chain Monte Carlo algorithms. A video-poster about some of this work can be found here. This research is carried out in collaboration with the groups of Erik Gauger (quantum theory) and Yoann Altmann (applied statistics).
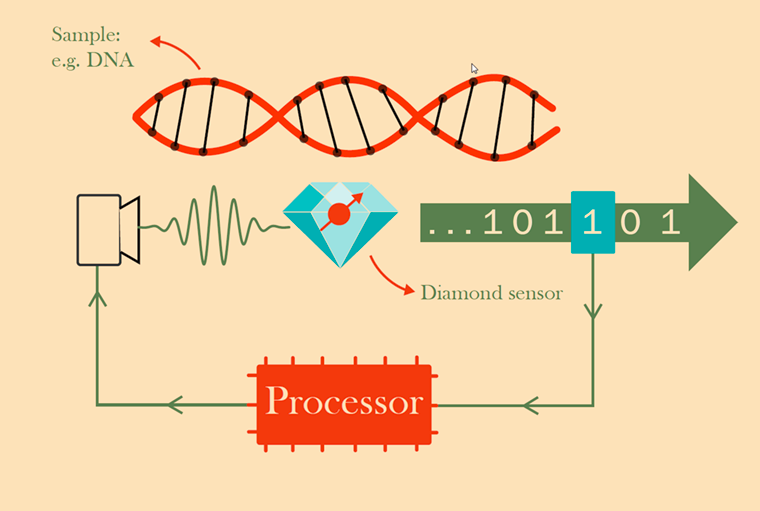
Quantum sensing of complex quantum materials. We have recently been awarded funding to set-up the Quantum Magnetometry Facility, comprising a unique set of instruments based on single-spin quantum sensing. Our goal is to apply quantum magnetometry to investigate magnetic fields and currents associated with quantum materials, in particular twisted 2D heterostructures. Stay tuned for more information!